This article has been reviewed according to Science X's editorial process and policies. Editors have highlighted the following attributes while ensuring the content's credibility:
fact-checked
peer-reviewed publication
trusted source
proofread
The most massive neutron stars probably have cores of quark matter
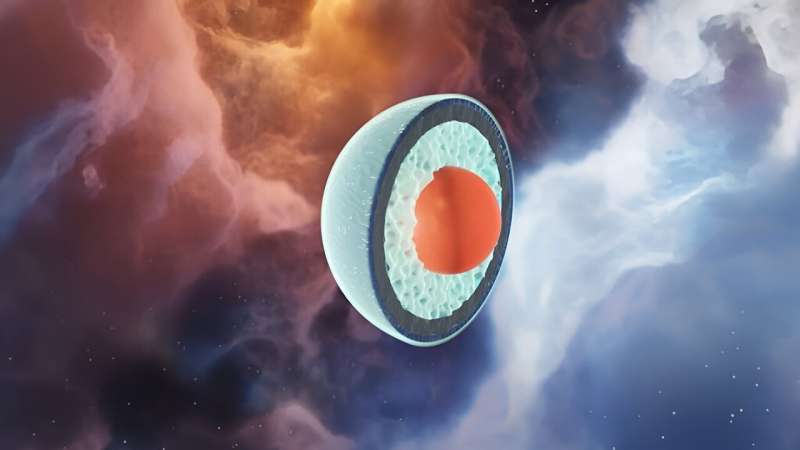
Atoms are made of three things: protons, neutrons, and electrons. Electrons are a type of fundamental particle, but protons and neutrons are composite particles made of up and down quarks. Protons have 2 ups and 1 down, while neutrons have 2 downs and 1 up. Because of the curious nature of the strong force, these quarks are always bound to each other, so they can never be truly free particles like electrons, at least in the vacuum of empty space. But a new study in Nature Communications finds that they can liberate themselves within the hearts of neutron stars.
Neutron stars are the remnants of large stars. They are a last-ditch effort to keep a stellar core from collapsing into a black hole. After all the nuclear fuel of a dense core is spent, the only thing that can counter gravity is the quantum pressure of neutrons. And that's where things get complicated.
The simple model of a neutron star holds that its core is filled with neutrons just on the edge of collapsing in on themselves. They may jostle against each other with tremendous energy, but they are still neutrons. The quarks within them are bound far too tightly for the neutrons to break apart. But some have argued that at this gravitational edge, neutrons can loosen up, allowing their quarks to flow together into a kind of quark soup. This would mean that neutron stars could have a dense quark core.
Unfortunately, we can't run experiments on neutron stars, nor can we create the kind of dense nuclear matter of a neutron star on Earth, but we do have some idea of how dense nuclear matter behaves through its equation of state. An equation of state is a way of calculating the bulk properties of a material, and for neutron stars that equation of state is known as the Tolman-Oppenheimer-Volkoff (TOV) equation. The only problem is that the TOV is an incredibly complex equation, and if you use it to calculate whether neutron stars have a quark core the answer you get is… maybe.
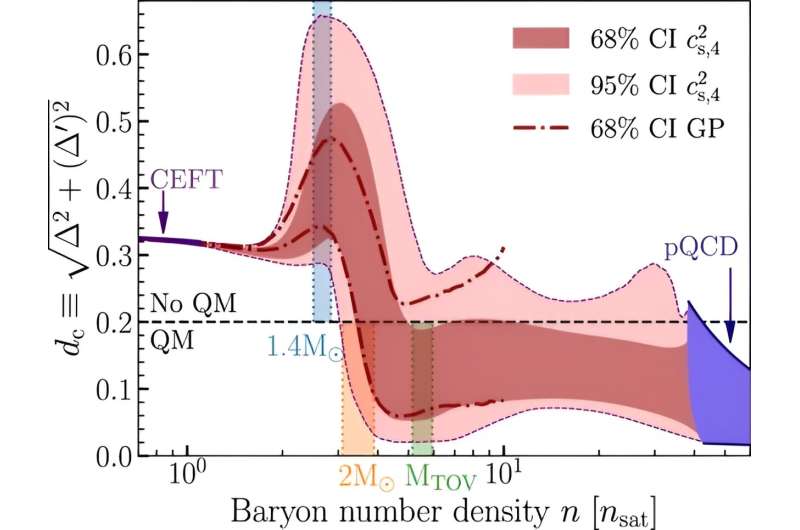
For this new study, the team took a different approach. Instead of churning through the equation of state calculations, they took observational data on the mass and size of neutron stars and applied Bayesian statistics. This statistical method looks at patterns of observation and extrapolates likely scenarios in a subtle but powerful way. In this case, if neutron stars have a quark core then they are slightly more dense than neutron stars without a quark core. Since small neutron stars likely don't have quark cores, while the most massive neutron stars likely do, a shift in the mass-density relation should show up in Bayesian analysis.
The team found that massive neutron stars, those with masses greater than two suns, have about an 80%–90% likelihood of having quark cores. It seems the real question is not whether quark stars exist, but rather where is the transition between quark stars and regular neutron stars.
To be fair, this analysis relied on a fairly small data sample. We currently don't know both the mass and radius of most neutron stars, but that will change over time. With more data, we should be able to pin down the critical phase shift between quark matter and dense neutron matter. But for now, we can be fairly certain that some neutron stars are much stranger than we had imagined.
Journal information: Nature Communications
Provided by Universe Today